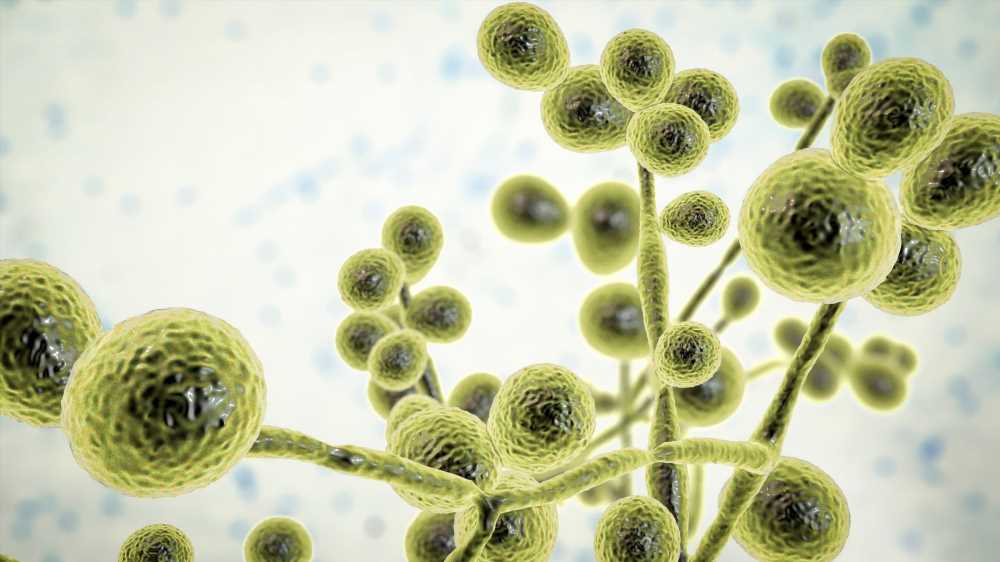
Understanding the mechanisms behind rising antifungal drug resistance
In a recent study published in npj Antimicrobials and Resistance, researchers addressed the issue of the rise of antifungal drugs-resistant infections and the advent of multidrug-resistant fungal pathogens. In addition, they described the strategies used by fungal pathogens to evolve resistance and the mechanism(s) governing the same.
To this end, they extensively reviewed recent literature on the mechanisms controlling resistance in emerging multidrug-resistant fungal pathogens, especially two species of the Candida genus, Candida auris, and Candida glabrata.
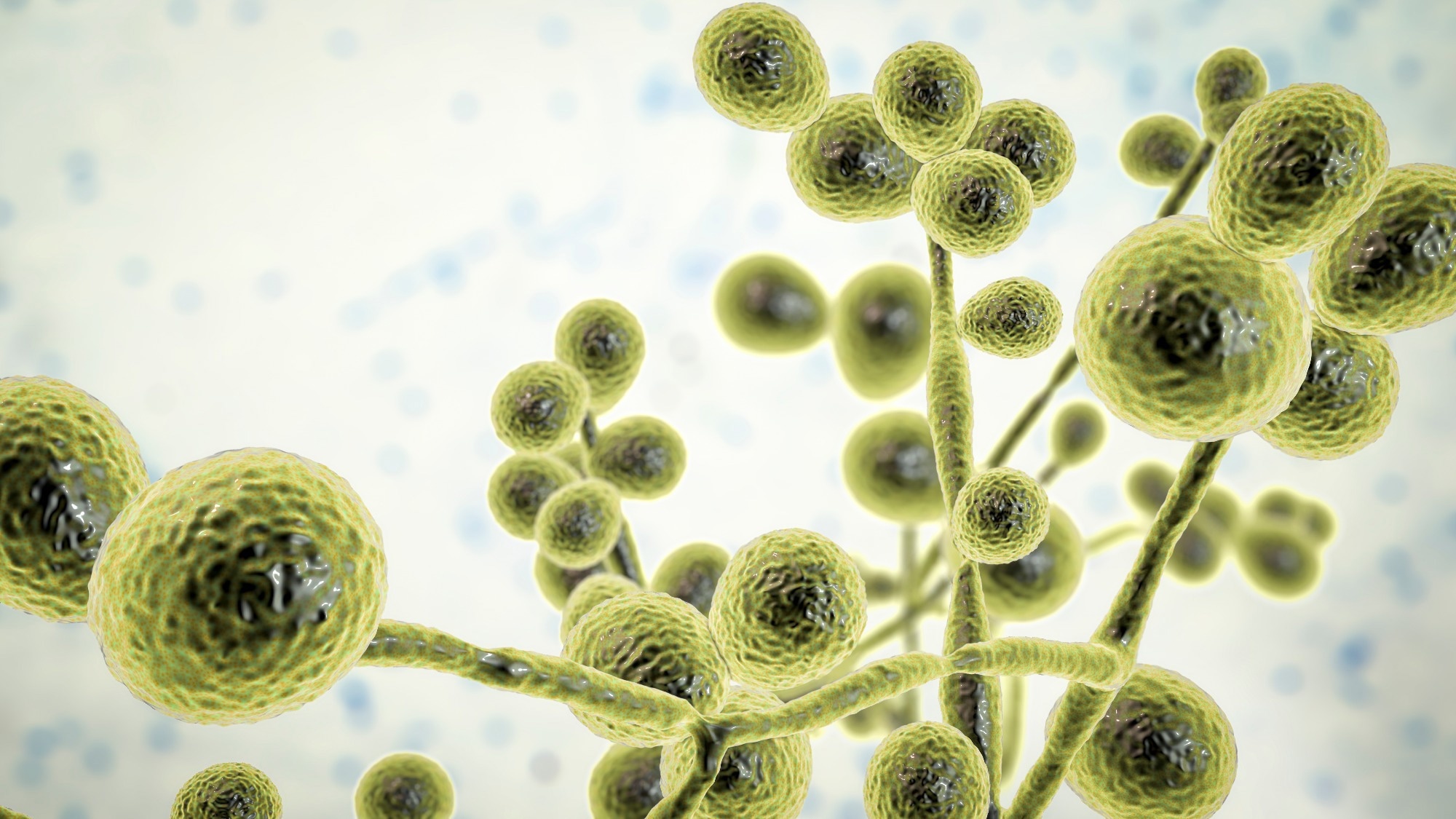
Background
The top three genera, Candida, Cryptococcus, and Aspergillus, cause the most systemic fungal infections. These opportunistic pathogens cause severe morbidity and mortality in immunocompromised individuals, claiming nearly 1.5 million lives annually.
For instance, Candida albicans cause life-threatening invasive candidiasis in immunocompromised people with mortality rates >40% even after treatment. Likewise, Aspergillus species causing invasive aspergillosis carry a mortality rate of 50% which can approach 100% if left undiagnosed or untreated.
Only three classes of drugs – polyenes, azoles, and echinocandins – are currently available to combat these lethal fungal pathogens. Amphotericin B, a polyene drug, is the treatment of choice for infections caused by C. neoformans. It exhibits broad-spectrum activity against many yeast and mold species but induces host toxicity too, which disfavors its wide clinical use.
Azoles, e.g., fluconazole, combat Candida species but disrupt the metabolism of other drugs by inhibiting the cytochrome P450. Echinocandins (e.g., caspofungin) have fungicidal and fungistatic activity in Candida and Aspergillus species but not C. neoformans.
Overall, the development of antifungal drugs has been slow-paced, thus, limiting the number of drugs available. Even these drugs pose high host toxicity, have unfavorable pharmacokinetics, and limited activity.
Clearly, as more multidrug-resistant fungal pathogens emerge, the current antifungal arsenal will not only become inadequate for clinicians but highly ineffective too. Thus, there is an urgent need to devise therapeutic strategies to combat pathogenic fungi, which are rapidly evolving drug resistance, increasing the threat to global human health.
Modes of acquiring antifungal drug resistance
Drug target alteration
Published literature describes over 140 unique sterol 14-demethylase (Erg11) amino acid substitutions in C. albicans, a structural analysis of which found that while some substitutions directly affected azole binding, those occurring on the proximal surface indirectly contributed to azole resistance. Some ERG11 mutations also occurred in azole-resistant clinical isolates of C. neoformans.
Unlike Candida, in A. fumigatus, mutation of cytochrome P450 14-α sterol demethylase (cyp51A) is a determinant for triazole resistance. Like Erg11, it catalyzes a demethylation step in ergosterol synthesis, the key sterol in the fungal cell membrane. Due to the excessive use of azole in the agriculture industry, the cyp51A gene mutations coupled with amino acid substitutions, such as TR34/L98H, are now widespread in the environment.
Perhaps, a recombination event between resistant and susceptible Aspergillus isolates has made this fungus a reservoir for drug-resistant clinical infections globally. Naturally, this organism feeds on organic debris in the soil as a saprotroph. Another mode of azole resistance acquisition is increasing drug target expression.
Fitness trade-offs disfavor the acquisition of resistance to the polyene in multiple fungal species. For the same reason, echinocandin resistance in clinical settings is rare for A. fumigatus.
Note that resistance to echinocandin frequently involves mutations in genes encoding the drug target, FKS1 and FKS2. These genes help in the synthesis of β-1,3-glucan, a core component of the fungal cell wall during vegetative growth and sporulation, respectively.
Fungi and humans share a close evolutionary trajectory which has limited the availability of fungus-specific targets for drug discovery. Further exacerbating this issue is the evolution of resistance to current antifungal drugs, especially azoles, and echinocandins, by target alteration.
Increased efflux
Resistance to azole therapy involved the upregulation of plasma membrane efflux pumps, which reduce drug accumulation within the target cells. Conversely, efflux-mediated resistance mechanisms play no role in adaptive resistance to echinocandins or polyenes, drug classes which are poor pump substrates. In addition, gain-of-function mutations in transcriptional activator genes, such as TAC1B, contribute to fluconazole resistance in C. auris.
Furthermore, overexpression of the ATP-binding cassette (ABC) transporters, Afr1 and AtrF, mediates azole resistance in C. neoformans and A. fumigatus, respectively. These observations highlight the need to target targeting drug efflux to overcome resistance.
Genomic plasticity
Fungal pathogens have remarkable genomic plasticity; accordingly, they alter their genomes rapidly via aneuploidy, loss of heterozygosity (LOH) events, and copy number variations (CNVs), which amplify large genomic regions. In addition, heteroresistance gave rise to highly azole-resistant fungal populations. A recent study also demonstrated horizontal gene transfer (HGT)-mediated antifungal resistance in A. fumigatus.
Cellular stress responses
Hsp90, a molecular chaperone regulating stress response circuitry, helps fungal pathogens acquire resistance to the azole and echinocandin drugs. Its key client proteins are calcineurin and protein kinase C (PKC).
In a recent study, researchers devised a tractable genetic system to show that depletion of HSP90 increases susceptibility to azoles and other cell stressors in C. neoformans.
Mutations, specifically, loss-of-function mutations in the Δ-5,6-desaturase gene, ERG3, have been identified in C. auris following echinocandin exposure, the first line of defense against invasive candidiasis.
Against echinocandins, C. albicans and A. fumigatus upregulate chitin, an essential fungal cell wall polysaccharide, which helps compensate for cellular stress induced by the inhibition of glucan synthase.
In C. albicans, PKC also regulates membrane stress responses in an Rlm1-dependent manner. It is a terminal transcription factor of the PKC-Mitogen-activated protein kinases (MAPK) cascade involved in fungal cell wall biogenesis.
Another downstream effector of the PKC-MAPK cascade, SBF, regulated the transcription of cell wall-related genes, including FKS2, under drug-induced cell wall stress.
Epigenetic regulation
Chromatin-based modifications are the most common epigenetic factors mediating drug resistance in fungal pathogens. In the early stages of resistance acquisition in fluconazole-resistant C. albicans isolates, studies have shown a marked upregulation of lysine deacetylases, histone deacetylase-A1 (HDA1), and reduced potassium dependency 3 (RPD3) expression.
Drug-induced or genetic inhibition of lysine deacetylases hampers the physical interaction between Hsp90 and calcineurin proteins needed to mount cellular stress responses, often resulting in azole resistance. In addition, lysine acetyltransferases, such as Gcn5, mediate resistance in Candida species.
Researchers are also exploring the role of post-translational modification in histone proteins and their impact on resistance to antifungals. In C. albicans, studies have implicated the SWI/SNF chromatin remodeling complex as a coactivator of fluconazole resistance.
Furthermore, studies have found that ribonucleic acid interference (RNAi)-dependent epimutations help acquire antifungal resistance via degradation of messenger RNAs of drug target genes, for instance, in Mucor circinelloides, the fungal species causing mucormycosis. It has attracted the attention of researchers to RNA-based therapeutics, especially for managing crop diseases caused by fungi.
Conclusions
Given the impact of fungi on food crop production and wildlife, the overwhelming rise in the prevalence of drug-resistant fungal pathogens poses a threat to global food security and the ecosystem.
The current study investigated the advancements in the area of antifungal drug research in recent years and made some important observations. First, they found new antifungal agents on the block with distinct modes of action, for example, olorofim, fosmanogepix, and ibrexafungerp.
Intriguingly, ibrexafungerp inhibits glucan synthesis like the echinocandins but uses a different mode of action. Secondly, they deciphered an alternative strategy to expand the antifungal armamentarium, i.e., combination therapy.
Besides drug optimization, high-throughput screening of compound libraries could help bolster the antifungal drug pipeline.
However, concerted efforts to develop a better mechanistic understanding of antifungal drug resistance are paramount to support the development of therapies for invasive fungal infections.
- Lee Y, Robbins N & Cowen LE (2023). Molecular mechanisms governing antifungal drug resistance. Npj. Antimicrob Resist 1, 5. doi: 10.1038/s44259-023-00007-2. https://www.nature.com/articles/s44259-023-00007-2
Posted in: Medical Science News | Medical Research News | Disease/Infection News | Pharmaceutical News
Tags: Agriculture, Amino Acid, Antifungal, Aspergillosis, Candida, Candida Albicans, Candida Auris, Candidiasis, Cell, Cell Membrane, Cell Wall, Chromatin, Compound, Cytochrome P450, Drug Discovery, Drugs, Evolution, Food, fungi, Gene, Gene Transfer, Genes, Genetic, Genomic, High-throughput screening, Kinase, Lysine, Membrane, Metabolism, mold, Mortality, Mucormycosis, Mutation, Pharmacokinetics, Potassium, Protein, Research, Ribonucleic Acid, RNA, RNAi, Stress, Therapeutics, Transcription, Yeast
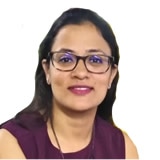
Written by
Neha Mathur
Neha is a digital marketing professional based in Gurugram, India. She has a Master’s degree from the University of Rajasthan with a specialization in Biotechnology in 2008. She has experience in pre-clinical research as part of her research project in The Department of Toxicology at the prestigious Central Drug Research Institute (CDRI), Lucknow, India. She also holds a certification in C++ programming.